Neuroscience has come a long way since the hand drawings of Ramón y Cajal. Innovative tools and technologies now allow scientists to capture the beauty of the brain in more ways than ever before. As part of the virtual 6th Annual BRAIN Initiative® Investigators Meeting, we hosted the second annual “Show Us Your BRAINs!” Photo and Video Contest. Presented below are six of the top contest entries and the scientists behind the microscope.
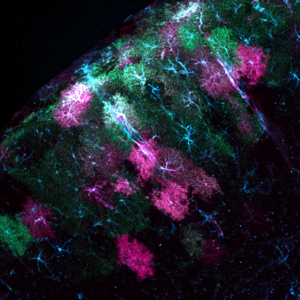
“Stargazer: Using light to study astrocytes”
Mosaic of astrocytes in the mouse visual cortex expressing a protein used to measure calcium flux (green) and a novel optogenetic tool designed to disrupt cell activity (ChromeQ; magenta). Starry astrocyte cytoskeletons are colored blue. Credit: Grayson Sipe, Massachusetts Institute of Technology (MIT), Cambridge, MA
Pictured here in blue, green, and magenta are astrocytes in the mouse visual cortex, the primary brain region that processes visual information. Astrocytes are star-shaped cells that were once thought to play only a supporting role in the nervous system. New technologies designed to visualize and manipulate astrocytes have allowed neuroscientists to learn more about these mysterious cells.
“Astrocytes represent a substantial cellular population in the brain, and yet we have a minimal understanding of what they do,” says Grayson Sipe, Ph.D., a postdoc at MIT and the artist behind this picture. Dr. Sipe’s photo was made possible by a BRAIN Initiative grant focused on inventing tools to study how astrocytes influence neuronal circuits in the cortex during visual and motor learning.
Dr. Sipe and his collaborators have developed novel tools to illuminate how these cosmic cells influence neural circuits. The green patches in his image are astrocytes genetically engineered to light up in response to calcium fluxes that flow through the cells, which may be triggered (or set off) by the activity of neurons and other cell types. By peering through a tiny glass window placed above the brain’s surface, Dr. Sipe can watch this activity in real time as mice are shown steady streams of visual stimuli. Magenta represents astrocytes filled with ChromeQ, a new genetically modified protein designed to disrupt (or “turn off”) astrocytes in response to light. By combining these two tools, he can simultaneously monitor and manipulate astrocyte activity as surrounding neural circuits process what a mouse sees.
“We now know that a single astrocyte can communicate with thousands of neuronal circuits and other cellular elements simultaneously,” says Dr. Sipe. “This suggests that the language of astrocytes in brain function is a complex and sophisticated convergence of many signals.”
We now know that a single astrocyte can communicate with thousands of circuits and cellular elements simultaneously. – Grayson Sipe, Massachusetts Institute of Technology (MIT)
Grayson Sipe earned his Ph.D. in Neurobiology from the University of Rochester in upstate New York. Currently, he is a postdoctoral fellow in Dr. Mirganka Sur’s lab at MIT. Learn more about his tool development projects by checking out the MIT Picower Institute for Learning and Memory news release featuring Grayson and Dr. Sur, or by connecting with him on Twitter (@gorsonsipe).
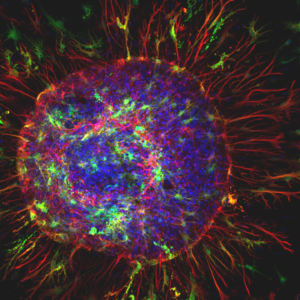
“Astro Sun”
Rat spinal cord astrocyte (blue and red) surrounded by toxic superoxide dismutase protein aggregates (green) known to underlie the neurological disorder amyotrophic lateral sclerosis (ALS). Credit: Karthik Krishnamurthy, Shashirekha Markandaiah, Brandie Morris Verdone, Davide Trotti, Piera Pasinelli, Thomas Jefferson University, Philadelphia, PA
“Astro Sun in Action”
Live footage of an astrocyte releasing toxic ALS-linked SOD-1 proteins (green) on to a healthy neighboring motor neuron (blue). Credit: Karthik Krishnamurthy, Shashirekha Markandaiah, Davide Trotti, and Piera Pasinelli, Thomas Jefferson University, Philadelphia, PA
Shown here are astrocytes grown in a dish from rat spinal cord tissue. They were captured by Karthik Krishnamurthy, Ph.D., a postdoc at Thomas Jefferson University, and his colleagues Drs. Davide Trotti and Piera Pasinelli. This research team studies the cellular and molecular basis of amyotrophic lateral sclerosis (ALS), or Lou Gehrig’s disease, a fatal and paralyzing disorder that damages motor neurons in the brain and spinal cord. Scientists suspect that toxic astrocytes may be to blame for some forms of ALS.
In contrast with Dr. Sipe’s cosmic photo of starry brain cells, this astrocyte (top; colored in red and blue) looks sinister. Astrocytes normally help support neuron health, but in ALS they undergo a deadly transformation caused by a mutation in a gene called superoxide dismutase-1 (SOD1). In addition to shifting astrocyte shapes, the mutations also cause the cells to release toxic substances that can harm nearby motor neurons. The green flecks in Dr. Krishnamurthy’s image are clumps of toxic SOD1 proteins surrounding an astrocyte. These dysfunctional astrocytes exacerbate motor neuron damage over time, contributing to ALS progression.
“We are just beginning to unravel how these supporting astrocytes turn out to be deadly neighbors to motor neurons”, says Dr. Krishnamurthy. Despite its malevolence, this astrocyte looks stunning. Dr. Krishnamurthy fondly calls his image ‘Astro Sun’ due to its resemblance to the Sun.
We are just beginning to unravel how these supporting astrocytes turn out to be deadly neighbors to motor neurons. – Karthik Krishnamurthy, Thomas Jefferson University
Dr. Krishnamurthy also used a power microscope to film in real time the release of toxic SOD1 proteins from a spinal cord astrocyte in a petri dish (bottom). The mutant SOD1 protein is colored green while astrocytes and neurons are blue.
In his video Krishnamurthy captures SOD1-carrying astrocytes damaging neighboring healthy neurons. “This provides further insights in to how the release of toxic proteins from astrocytes might be a trigger for the spreading of toxicity in ALS,” says Dr. Krishnamurthy. By closely studying mutant astrocyte toxicity, Dr. Krishnamurthy and his colleagues hope to better understand the molecular basis of ALS and inform drug therapies designed to halt disease progression in humans.
Karthik Krishnamurthy earned his Ph.D. in biophysics from the National Institute of Mental Health and Neurosciences in Bangalore, Karnataka, India. Currently, he is a postdoctoral fellow in Dr. Piera Pasinelli’s lab at the Thomas Jefferson University Weinberg ALS Center. Learn more about his research by connecting with him on Twitter (@cellsandme).
“The Symphony of Dancing Stars”
Real-time imaging of rat cortical neurons in a cell tissue plate stimulated three times by the neurochemical glutamate. Neurons are multi-colored and surrounding cells (such as astrocytes and other glia) are blue. Credit: Elnaz Khezerlou, Louisiana Tech University, Ruston, LA
This video shows a ‘symphony’ of neural activity. It was filmed by Elnaz Khezerlou, a doctoral student in neuroscience at Louisiana Tech University. She works in a lab that uses advanced imaging methods to study the activity of brain cells in real time. The lab is part of a BRAIN Initiative project focused on using these tools to uncover the circuit dynamics behind epileptic seizures and memory.
For this video Ms. Khezerlou used a special camera to film waves of calcium flowing through rat cortical neurons in a cell tissue plate. Each cell is filled with a fluorescent probe designed to shine during a calcium wave. The video captures three synchronized bursts of activity in response to the excitatory neurochemical glutamate. The bursts are reminiscent of neural feedback loops seen in the human brain, such as during an epileptic seizure.
“This shows us that in the brain, changes are happening in time and in space,” says Ms. Khezerlou.
She also studies how neighboring star-shaped astrocytes, which are hidden in the video, may help synchronize neural firing patterns by slowing (or preventing) calcium from flowing out of neurons during a process called negative feedback. A break down in this process may underlie the seizures observed in some cases of epilepsy and brain cancer.
Elnaz Khezerlou holds an undergraduate degree in Chemical Engineering and a master’s degree in Biotechnology from Islamic Azad University, Science and Research Branch, in Tabriz, Iran. Currently, she is a Ph.D. student in Dr. Mark DeCoster’s lab at Louisiana Tech University.
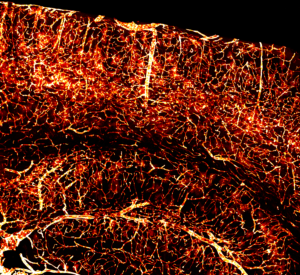
“Brain in Flames”
An intricate network of blood vessels in the mouse cortex. A newly engineered virus, AAV-PHP.V1, was used to target and encode fiery fluorescent proteins (tdTomato; red) in endothelial cells throughout the brain.
“Brain Vasculature in 3D”
Thousands of pictures of the mouse brain were stitched together to create this stunning 3D video of brain vasculature. Photo and video credit: Sripriya Ravindra Kumar, California Institute of Technology, Pasadena.
This fiery picture of mouse brain blood vessels was captured by Sripriya Ravindra Kumar, Ph.D., a scientist and Director of AAV Engineering at the Caltech Beckman Institute CLOVER Center. She works with a research team funded by the BRAIN Initiative to develop a ‘toolbox’ of viruses designed to visualize and study various cell types and circuits throughout the brain.
Dr. Kumar re-engineers viruses to safely deliver gene therapy genetic material to specific cells in different parts of the brain and body. Here she debuts a new virus called AAV-PHP.V1, which loads genes into blood vessel cells. In this case, the genes contained instructions for making red fluorescent proteins.
The virus can also cross the blood-brain barrier, which is notoriously difficult for viruses to penetrate, says Dr. Kumar. This means that it reaches the brain by traveling through the bloodstream after a simple intravenous injection, making it easier to study and treat brain disorders. According to Dr. Kumar, the virus is extremely useful because vascular cells are implicated in many neurological conditions, including Alzheimer’s disease and stroke.
Kumar also provided a 3D tour of the mouse brain. “The brain is a highly vascularized organ and being able to visualize this complex vasculature in a 2D and 3D is very fascinating,” says Kumar.
For the video Kumar used a relatively new method called immunolabeling-enabled three-dimensional imaging of solvent-cleared organs (iDISCO). This lengthy tissue-clearing process involves the use of fat-dissolving solvents to make the brain clearer to see under the microscope. The brain is then flooded with fluorescently labeled antibodies designed to stain cells infected with the newly created virus. After staining them, the blood vessels light up under a high-powered microscope. Thousands of these pictures were ‘stitched’ together to visualize the vessels in 3D.
“Given that vasculature is such a dense network, we wanted to show that the virus works across the whole brain,” says Dr. Kumar.
According to Kumar, her video represents several modern tools in neuroscience that make it possible to see features of the brain in such detail. “Maybe seven or eight years ago, this would not be possible,” she says.
Maybe seven or eight years ago, this would not be possible. – Sripriya Ravindra Kumar, California Institute of Technology
Dr. Kumar’s doctoral dissertation started out as a viral engineering project. But as it progressed, scientists in other disciplines took notice. “It was unexpected that this would evolve into something that is so broad and of interest to so many people in neuroscience,” says Dr. Kumar. Now that she has showcased the virus’ ability to beautifully label entire networks of blood vessels, she hopes to widely share her lab’s new tool with research community.
Sripriya (Priya) Ravindra Kumar received her B.Tech degree from Anna University in India and M.S. degree in Medical Biotechnology from the University of Illinois at Chicago. She earned her Ph.D. from Caltech and is now the Director of AAV Engineering at Caltech’s Beckman Institute CLOVER Center run by Dr. Viviana Gradinaru. Learn more about Priya’s viral engineering projects by reading her recent Nature Methods paper or by connecting with her on Twitter (@KumarSripriya).
Check out the first, second, and third place winners of the “2020 Show Us Your BRAINs!” Contest here. Feeling creative? Next year’s photo and video contest will open for submissions in April 2021.
Other articles in this series include: