As part of the virtual 6th Annual BRAIN Initiative® Investigators Meeting, we hosted the second annual Show Us Your BRAINS! Photo and Video Contest. In the third article of this series, we feature five of the top entries and the scientists (and artists) behind them. The photos below are also part of the 2021 BRAIN Initiative Calendar – available for digital download.
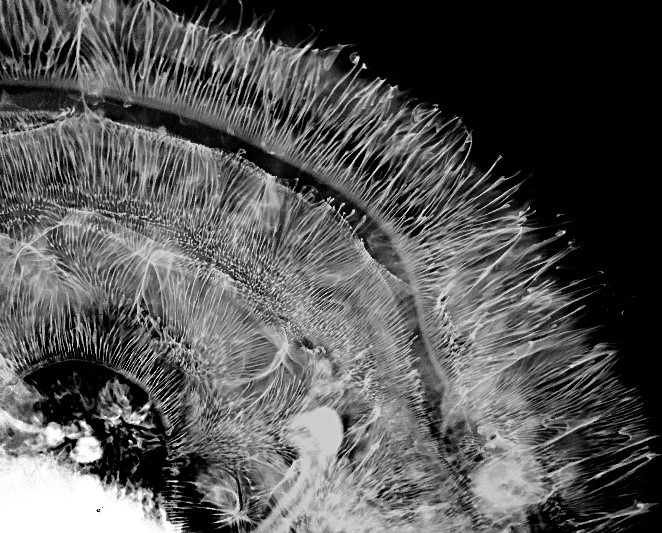
3rd Place Winner. “The Ephemeral Hippocampus”
This exquisite ‘hippocampus’ is not a brain, but an image during the initial steps of a printmaking technique. Credit: Tallie Z. Baram, Jeremy Barry, and Joan Morris, University of California – Irvine, Irvine, CA, © 2017 Joan Morris.
In this photo, art and science collide. To a neuroscientist this may look like a mouse or rat hippocampus, a brain region important for learning and memory. But this image is actually a waterdrop captured during a printmaking technique called intaglio. The method used here involves the diffusion of dye in water to create abstract patterns and shapes.
The photo was created by Joan Morris, a renowned artist and fabric dyer, who shared the photo with her neuroscientist colleagues. They were immediately struck by its beauty and uncanny resemblance to a slice of brain tissue.
“The pattern of hippocampal cell layers and dendrites during sensitive stages of brain development is not unlike the movement of the dye in Joan’s intaglio process,” says Tallie Z. Baram, M.D., Ph.D., a pediatric neurologist and neuroscientist at the University of California, Irvine, who entered the photo into the contest.
The pattern of hippocampal cell layers and dendrites during sensitive stages of brain development is not unlike the movement of the dye in Joan’s intaglio process. – Tallie Z. Baram, University of California, Irvine
Dr. Baram and her research team study how seizures and trauma in early life impact the development of neural circuits in the hippocampus. In children, these events can disrupt the normal growth and activity of the circuits, leading to serious memory problems later in life. Similarly, the patterns formed by the dyes in this photo are extremely vulnerable to perturbations, especially early on in the artistic technique.
“We work on the beauty and fragility of developing circuits,” says Dr. Baram. By using a variety of novel neuroscience techniques to study the molecular, cellular, and behavioral underpinnings of brain development, she hopes to inform therapies for memory deficits and neuropsychiatric conditions, such as depression.
Tallie Z. Baram is a professor of pediatrics, neurobiology, neurology and physiology/ biophysics, and the Danette Shepard Professor of Neurological Sciences at the University of California, Irvine. Learn more about her research on how early-life events impact the developing brain by visiting the Baram lab website.
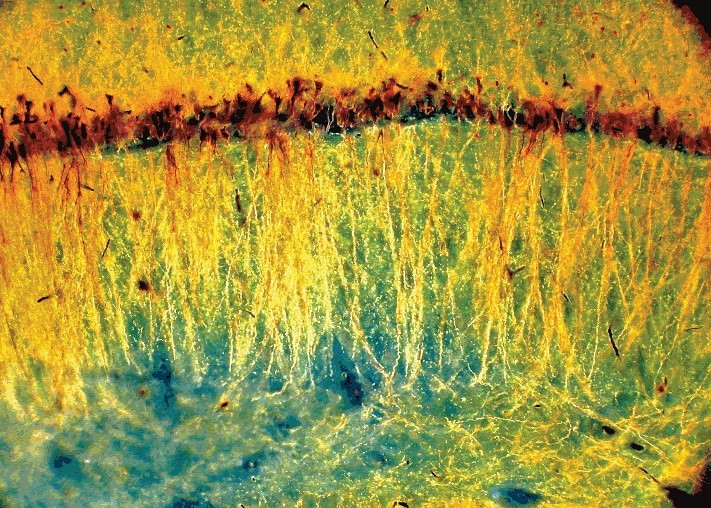
“Forest Gold”
Pyramidal neurons in the CA1 layer of the rat hippocampus. The ‘somatodendritic tree’ of each neuron (gold) was labeled using the Gallyas method, an improved Golgi silver staining technique, and illuminated with dark-field microscopy. Credit: György Buzsáki, New York University, New York, NY.
Shown here are neurons in the rat hippocampus. The image was submitted by György Buzsáki, M.D., Ph.D., a professor of neuroscience at the New York University School of Medicine. He studies the brain basis of learning and memory by examining patterns of neural activity or ‘neural syntax’ in the hippocampus. Dr. Buzsáki and his collaborators are using the latest technologies in neuroscience to create a computational model of the hippocampus, including its cell types and networks. Contrary to his recent work, Dr. Buzsáki appreciates the beauty of long-lost neuroscience techniques.
More than a century ago, Camillo Golgi, an Italian scientist revolutionized brain science. His silver staining technique – coined the Golgi method – made it possible to see the intricate branches of single cells among a dense forest of neurons. In this photo, neurons and their entire ‘somatodendritic trees’ were stained using the Gallyas method, a modified Golgi technique. Dendrites are branching structures that extend from the soma (cell body of a neuron) and receive signals from other neurons. After staining, the tissue was imaged using a dark-field microscope, a technology that has also existed since Golgi first saw neurons in brain tissue.
“Dark-field illumination is a long forgotten method of Old World anatomists. The dark field can bring about spectacular colors, such as gold, and as such is a great antidote for today’s RGB colors typical of multicolor immunostaining,” says Dr. Buzsáki.
Dark-field illumination is a long forgotten method of Old World anatomists. The dark field can bring about spectacular colors, such as gold, and as such is a great antidote for today’s RGB colors typical of multicolor immunostaining. – György Buzsáki, NYU School of Medicine
These early techniques have some advantages over modern staining and microscopy methods. For instance, in this image, a trained eye can separate healthy neurons from damaged ones and quantify the position of cell bodies (orange) within certain layers of the hippocampus.
György Buzsáki is a Professor of Neuroscience at the NYU School of Medicine. Learn more about his research on the ‘syntax of the brain’ by visiting the Buzsáki lab website or by reading his new book The Brain from Inside Out.
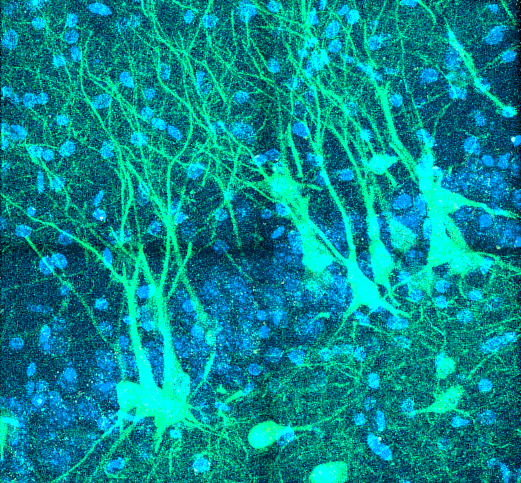
“Diverse CA3 Morphology”
Neurons in the CA3 region of the hippocampus expressing a protein used to monitor the activity of cell bodies and their branching dendritic trees (GCaMP6f; green). Credit: Jason Moore and Jayeeta Basu, New York University, New York, NY.
Pictured here are neurons in the CA3 region of the hippocampus, a subregion of the brain important for spatial memory. The image was captured by Jason Moore, Ph.D., a postdoctoral fellow at the New York University School of Medicine. He works in a lab that uses advanced techniques in rodents to study how neural circuits control memory. The lab is funded by the BRAIN Initiative to use these tools to uncover how distant brain regions interact with single neurons and their dendrites in the hippocampus during memory formation.
The fluorescent green cells shown here are neurons labeled with a genetically engineered protein called GCaMP6f. The protein is designed to light up in response to waves of calcium that flow during neural activity. By using a powerful microscope, Dr. Moore can monitor the activity of these neurons and their tree-like dendritic arbors as rats navigate around an apparatus designed to test their memory.
“Substantial amounts of energy and genetic programming go into creating and maintaining these long, elaborate dendritic trees. We believe they must endow neural circuits with abilities they wouldn’t otherwise have,” says Dr. Moore.
Substantial amounts of energy and genetic programming go into creating and maintaining these long, elaborate dendritic trees. We believe they must endow neural circuits with abilities they wouldn’t otherwise have. – Jason Moore, NYU School of Medicine
Dendrites have a diverse morphology, or come in all shapes and sizes, making them difficult to study and mathematically model. But with the advent of new tools and approaches to monitor their activity, Dr. Moore and his colleagues can shine light on the hidden contributions of dendrites to neural circuits.
Jason Moore earned his Ph.D. in neuroscience from UCLA where he refined a technique to record from dendrites in freely moving animals. Currently, he is a postdoctoral fellow in Dr. Jayeeta Basu’s lab at the NYU School of Medicine.
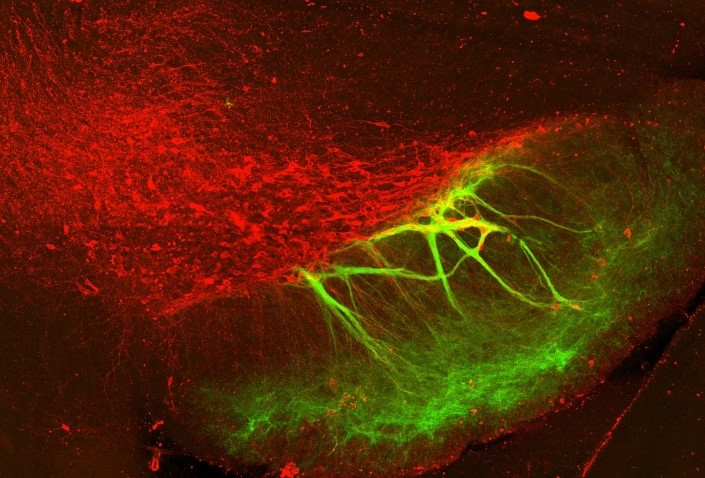
“Dopamine Lightning”
Axons of inhibitory neurons in the striatum (green) wrapping around dendrites of midbrain dopamine neurons (red). These unique connections are called ‘striosome-dendron bouquets.’ Credit: Rebekah Evans, National Institute of Neurological Disorders and Stroke (NINDS), Bethesda, MD.
Pictured here in green are axons, fibers that send information to other neurons, entwined with dopamine-producing neurons in a mouse brain tissue slice. The image was captured by Rebekah Evans, Ph.D., an assistant professor at Georgetown University. Dr. Evans recently received a BRAIN Initiative grant to apply computational, electrophysiological, and imaging techniques to dissect the neural circuits affected by neurodegenerative disorders, such as Parkinson’s disease.
These unique connections, called striosome-dendron bouquets, are found in the basal ganglia, a network of neurons important for decision-making, emotion, and movement. Originally named by Dr. Anne Graybiel after their bouquet-like appearance, the striosome fibers shown here act like a set of brakes by sending calming signals to nearby dopamine neurons. Initially, because they only touched the dendrites of these cells, Dr. Evans and her colleagues didn’t think much of these seemingly weak connections.
“Looking at the image, you may guess that the two structures barely interact with one another. But striosomal axons can exert powerful control over whole dopamine neurons, completely shutting down their activity,” says Dr. Evans, who made this surprising discovery. In this way, striosomes can control the timing of neural computations in the brain important for learning and motivation.
Rebekah Evans earned her Ph.D. in neuroscience from George Mason University and was a postdoc in Dr. Zayd Khaliq’s lab at the National Institute of Neurological Disorders and Stroke. Currently, she is an assistant professor at Georgetown University. Learn more about her research on neural circuit dynamics by visiting her lab website or by connecting with her on Twitter (@cellularscale).
“In Sync”
Synchronized neural and kinematic, or body movement, signals from an individual with an adaptive deep brain stimulation (DBS) implant used to treat Parkinson’s disease. Credit: Johanna O’Day, Kevin Wilkins, and the Bronte-Stewart lab, Stanford University, Palo Alto, CA.
This video represents the future of deep brain stimulation (DBS), a pacemaker-like brain implant used to remedy Parkinson’s disease. It was filmed by Johanna O’Day, Ph.D. candidate, and her colleagues at the Stanford University School of Medicine. She is part of a research team that is developing next-generation DBS devices. They are funded by the BRAIN Initiative to conduct the first-ever clinical studies of a self-tuning DBS device that responds to abnormal gait, or walking abnormalities, in people with Parkinson’s disease.
The person shown here has Parkinson’s disease and volunteered to be a part of the research studyHe uses DBS to treat his tremors, gait problems, and other motor symptoms caused by the disease.
DBS involves surgically implanting metal wires called electrodes deep into certain areas of the brain (top right image). The electrodes send electrical impulses that modulate brain activity. The level of stimulation is controlled by a medical device similar to a pacemaker that is placed under the skin in the chest. This man has electrodes in his subthalamic nucleus, a brain region commonly targeted with DBS. MRI neuroimaging was used to check the position of his electrodes.
Developed by Helen Bronte-Stewarther team of neuroscientists and engineers, this DBS device is adaptive, or self-tuning, meaning that it can adjust stimulation levels in response to brain activity.
The participant’s leg movements were captured by motion trackers similar to Fitbits, which were synchronized with his brain activity (left image). Eventually, the research team hopes to develop a device that responds to freezing and problems with walking in real-time. The hope is that this version of DBS will greatly help Parkinson’s disease patients move more naturally and improve the quality of their lives.
Johanna O’Day received her master’s degree in bioengineering from Stanford University, where she is currently pursuing a Ph.D. in bioengineering. She works in the Bronte-Stewart and Delp labs. Learn more about her research on biomechanics by following her on Twitter (@Johanping) or by checking out her story-telling initiative, the Parkinson’s Story Exchange.
Feeling inspired? This year’s contest opens for submissions on April 1, 2021. Check it out here.
Other articles in this series include: