As part of the virtual 6th Annual BRAIN Initiative® Investigators Meeting, we hosted the second annual Show Us Your BRAINs! Photo and Video Contest. In this article, we highlight five of the top entries and the scientists who created them. These photos are also featured in the 2021 BRAIN Initiative Calendar – available for digital download.
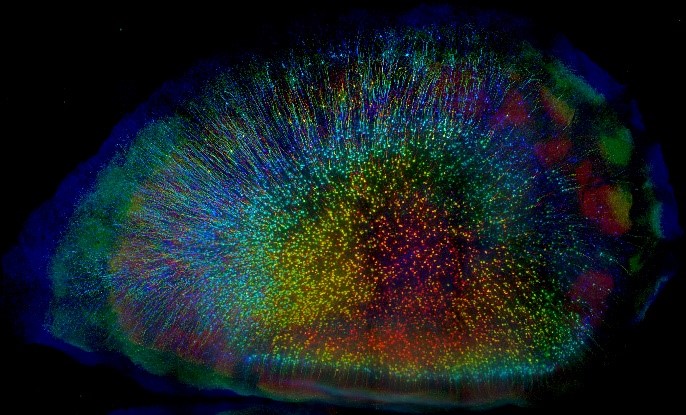
1st Place Photo Winner. “Cortical Forest” Mouse cortical neurons labeled with a fluorescent protein (eYFP). CLARITY, a tissue-clearing process, was used to make an entire brain transparent. Each layer of neurons is color coded to create a 3D view. Credit: Linus Manubens-Gil and Jim Swoger, Centre de Regulació Genòmica and EMBL Mesoscopic Imaging Facility, Barcelona, Spain.
Pictured here are cortical neurons in a mouse brain. This winning image was captured by Linus Manubens-Gil, Ph.D., a neuroscientist at South East University – Allen Institute Joint International Center for Brain Science and Jim Swoger, Ph.D., head of the Barcelona EMBL Mesoscopic Imaging Facility. As a postdoc at the Centre de Regulació Genòmica, Dr. Manubens-Gil and his colleagues were funded by the BRAIN Initiative to create user-friendly, computer programs to help neuroscientists understand how the shapes of single neurons relate to connections in larger brain networks.
This brain is from a mouse genetically engineered to express a fluorescent protein called eYFP in cortical neurons, which makes the cells glow under a microscope. Dr. Manubens-Gil first used a fat-stripping tissue preparation method called CLARITY to make the brain transparent and neurons easier to see. He then worked closely with Dr. Swoger to optimize a powerful new fluorescence microscope to image the neurons layer-by-layer in super high-resolution. Lastly, the images were combined to create a multicolored 3D view of a ‘cortical forest.’ Each neuron is color coded based on its depth.
“Recent advancements in tissue prep techniques and fluorescence microscopy have revealed unprecedented amounts of high-resolution information about brain structure and connectivity,” says Dr. Manubens-Gil.
Now, scientists can precisely study the branching patterns of single neurons and the topology of large brain networks, all in the same brain. Studying the brain across scales is important for understanding Down syndrome and other cognitive disorders accompanied by abnormal brain connectivity.
“This image shows very beautifully how tissue clearing techniques are bridging scales de facto,” says Dr. Manubens-Gil, who is statistically analyzing some of the world’s largest datasets of neural morphology to figure out how single neurons relate to neuronal networks. “Ultimately this will allow us to pinpoint fundamental rules for the healthy and faulty wiring of brain circuits.”
This image shows very beautifully how tissue clearing techniques are bridging scales de facto. Ultimately this will allow us to pinpoint fundamental rules for the healthy and faulty wiring of brain circuits.
– Linus Manubens-Gil, South East University – Allen Institute Joint International Center
Linus Manubens-Gil received his Ph.D. in biomedicine from Centre de Regulació Genòmica in Dr. Mara Dierssen’s lab, where he also completed his postdoc. Currently, he is the Group Head of systems neuroscience at the South East University – Allen Institute Joint International Center in Nanjing, China. To learn more about these projects connect with Linus (@linus_mg) or EMBL MIF (@BarcelonaMif) on Twitter.
1st Place Video Winner. “Journey Through a Mouse Brain”
3D tour of an optically cleared mouse brain. Credit: Sunil Gandhi, Ricardo Azevedo, and Damian Wheeler, University of California, Irvine and Translucence Biosystems, Irvine, CA.
This video of neurons in a mouse brain was created by Sunil Gandhi, Ph.D., Damian Wheeler, Ph.D., and Ricardo Azevedo, M.D./Ph.D. candidate, neuroscientists and founders of Translucence Biosystems. This biotechnology company provides researchers and clinicians with fast, reliable 3D analysis of biological samples. Dr. Wheeler and his team have a BRAIN Initiative grant to develop new tools and technologies to collect high-resolution whole brain snapshots of neural activity in 3D.
Here we travel from neurons in the cortex down a bundle of axons called the corticospinal tract, an intricate structure connecting the cortex to the spinal cord, and on to deeper brain regions. This video tour was created by combining the latest tissue-clearing and histological methods, advanced imaging technology, and 3D reconstruction data processing algorithms. With this toolset, scientists can see snapshots of neuronal activity and far-reaching circuits that span the entire brain.
“Now we can see intact, long-range circuits in the whole mouse brain in their full 3D complexity,” says Dr. Gandhi, who uses this tool to study how the visual system develops. “There is no doubt that this new technology will produce exciting new discoveries about the organization of the brain.”
There is no doubt that this new technology will produce exciting new discoveries about the organization of the brain.
– Sunil Gandhi, University of California, Irvine, Translucence Biosystems
Damian Wheeler earned his Ph.D. in physiology from McGill University and was a postdoc at Stanford. He then worked in drug discovery for nine years at Dart NeuroScience. In 2018, he teamed up with Dr. Sunil Gandhi and Ricardo Azevedo to form Translucence Biosystems. Learn more about how Translucence is revolutionizing next-generation histology by visiting their website.
“Retro ACC”
3D view of neural connections to the anterior cingulate cortex (ACC) in a mouse brain. A virus designed to travel backward from axon terminals to cell bodies of excitatory neurons was used to visualize the ACC network. Credit: Ron Refaeli, Hebrew University of Jerusalem, Jerusalem, Israel.
Shown here are neural connections of the mouse anterior cingulate cortex (ACC), a brain area known for orchestrating attention, impulse control, and decision-making. Thanks to new techniques designed to map and modulate neural circuits, scientists have recently found that the ACC also interacts with other brain regions to control long-term memory.
“The ACC and its connections to the hippocampus, a deep brain region needed for memory consolidation, are tightly related to memory formation and retrieval,” according to Ron Refaeli, a doctoral student at the Hebrew University of Jerusalem and artist behind the video. Mr. Refaeli works in a lab that uses modern neuroscience techniques to investigate the brain circuitry of long-term memory in mice.
To figure out how the ACC and hippocampus work together, Mr. Refaeli must first map their connections. For this video he used a virus engineered to safely map the connections between neurons. After injecting the virus into the ACC (white glow) it travels retrogradely, or backwards from axon terminals to cell bodies. Once the virus reaches the cell bodies it delivers genes that code for fluorescent proteins, making each ACC-projecting neuron and its pathway glow red under a microscope. Mr. Refaeli used CLARITY tissue-clearing to make the brain transparent and connections visible. Using computer software, hundreds of images were combined to create a 3D view.
Armed with a detailed map of ACC connectivity, Mr. Refaeli can now explore the role of specific circuits in long-term memory.
Ron Refaeli is a neurobiology Ph.D. student in Dr. Inbal Goshen’s lab at the Hebrew University of Jerusalem. Learn more about his research and the tools he uses to study memory by visiting the Goshen Lab website.
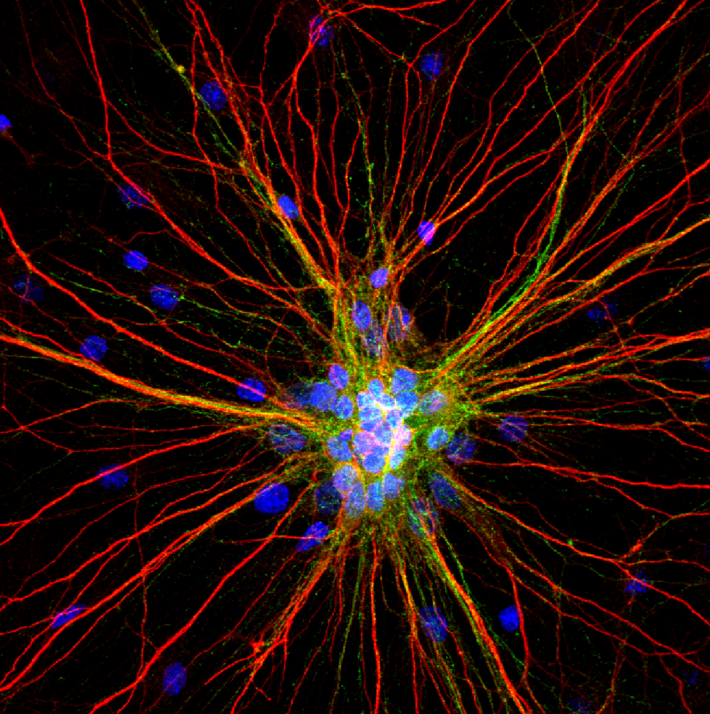
2nd Place Winner. “Radiating Neurons”
A cluster of 4-week-old cortical neurons stretching their neurites far and wide. The loss of connections formed by dendrites (red) and axons (green) in motor circuits is a hallmark of amyotrophic lateral sclerosis (ALS) and other neurodegenerative diseases. Credit: Karthik Krishnamurthy, Shashirekha Markandaiah, Davide Trotti, and Piera Pasinelli, Thomas Jefferson University, Philadelphia, PA.
Pictured here are 4-week-old rat cortical neurons grown in a dish. This image was captured by Karthik Krishnamurthy, Ph.D., a postdoctoral researcher at Thomas Jefferson University, and his colleagues Shashirekha Markandaiah, Davide Trotti, Ph.D., and Piera Pasinelli, Ph.D. This team studies the cellular and molecular basis of amyotrophic lateral sclerosis (ALS), or Lou Gehrig’s disease, an often fatal paralyzing disorder that damages motor neurons in the brain and spinal cord.
Also known as neurites, the red branches are dendrites and the wispy green branches are axons. Dendrites receive signals from other neurons and transmit them to the cell body, and axons send signals. Motor neurons can stretch their axons long distances in the nervous system. In the human brain, they project down from the cortex to the spinal cord, which then transmits nerve signals to muscles throughout the body. In ALS, motor neurons progressively break down and die, leading to muscle weakness, atrophy, and paralysis.
“Hyperexcitable”
Live video of a 10-day-old cortical neuron in a dish exposed to glutamate showing a hyperexcitable response. A genetically encoded calcium probe called GCaMP6 was used to measure calcium flux, a proxy for neural activity. Credit: Karthik Krishnamurthy, Davide Trotti, and Piera Pasinelli, Thomas Jefferson University, Philadelphia, PA.
Scientists believe that high levels of the neurochemical glutamate may be linked to motor neuron death. Normal levels of glutamate are important for natural communication between neurons, but too much of it can be toxic. When a neuron is hyperexcitable or overly activated, like the one filmed here, it releases excessive glutamate and kills downstream neurons.
For this video, researchers used a high-powered microscope to capture the activity of a neuron labeled with a genetically encoded calcium probe called GCaMP6. The fluorescent probe is designed to shine bright during calcium flow, which is used as a readout of neural firing (red line).
“Following a period of hyperexcitability, neurons follow a destructive path of degeneration, ultimately losing communication with each other,” says Dr. Krishnamurthy.
Interestingly, scientists believe that hyperexcitability of cortical neurons may emerge early in ALS. This may trigger the progressive destruction of motor neurons. By studying the cellular and molecular basis of neuronal death, Dr. Krishnamurthy and his colleagues hope to figure out ways to slow down or halt the progression of ALS and other neurodegenerative diseases.
Karthik Krishnamurthy earned his Ph.D. in biophysics from the National Institute of Mental Health and Neurosciences in India and was a research fellow at Johns Hopkins University before joining the Jefferson Weinberg ALS Center as a postdoc. Shashirekha Markandaiah earned her master’s degree in biochemistry from Kuvempu University, India and worked at the Bigtec Labs. Currently she is the laboratory coordinator at the Weinberg ALS Center where she studies the mechanisms by which glial cells contribute to ALS.
Check out the other winners who placed in the 2020 Show Us Your BRAINs! contest here. Feeling creative? This year’s BRAIN Initiative Investigators Meeting photo and video contest will open for submissions in April 2021.
Other articles in this series include: